49 Congreso Internacional del Americanistas (ICA) |
|
Quito Ecuador7-11 julio 1997 |
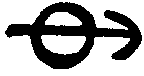
Giulio Bigazzi, Mauro Coltelli, Julio Cesar Hadler Neto and Ana Maria Osorio Araya
Provenance Studies of Obsidian Artefacts Using the Fission-Track Analysis in South America: an Overview
Giulio Bigazzi1, Mauro Coltelli2, Julio Cesar Hadler Neto3 and Ana Maria Osorio Araya4
1C.N.R. Istituto di Geocronologia e Geochimica Isotopica, Via Cardinale Maffi 36, 56127 Pisa, Italy.
2C.N.R. Istituto Internazionale di Vulcanologia, Piazza Roma 2, 95123 Catania, Italy.
3Dep.to Raios Cosmicos, Instituto de Fisica, Universidade Estadudal de Campinas - UNICAMP
C.P. 6165, 13081 Campinas SP, Brazil.
4Dep.to de Ciências Ambientais, Universidade Estadual Paulista - UNESP
C.P. 957, 19060 Presidente Prudente SP, Brazil
1. Introduction
Provenance identification of natural sources of raw material used by prehistoric men provides archaeologists a peculiar opportunity of detecting trade routes and cultural interactions.
Obsidian was widely used for tool-making during prehistoric times. This natural glass is an ideal material for correlation of artefacts with potential sources, because (1) it was recognised in the form of lava flows, domes or as a component of pyroclastic products in distinct volcanic areas and (2) its physical and/or chemical properties appear to vary according to the occurrence of origin. For these reasons, provenance studies of obsidians is among the most popular archaeometric research subjects.
Among the several approaches used for characterisation and discrimination of volcanic glass, chemical composition studies by major elements and/or trace elements analyses appear the most widespread techniques.
Since early sixties the induced tracks of 235U, produced by irradiation with thermal neutrons in a nuclear reactor, were revealed by chemical etching in several minerals and observed under an optical microscope (Price and Walker, 1962). These authors also observed natural tracks (named fossil or spontaneous tracks) in non-irradiated samples (Price and Walker, 1963): it was realised that insulating solids store memory of the 238U spontaneous fission events occurred in their interior during geological times. As fission occurs at a constant rate during time, the number of tracks present in a sample is proportional to its U-content and to the time elapsed since track recording started. Upon this principle is based the fission-track dating method, that was widely applied during the last 30 years especially for geological studies. For an exhaustive overview on fission-track dating and its application, see the recent book by Wagner and Van den haute (1992).
Chemical etching of a polished surface of a sample produces a track areal density r that can be measured by a counting procedure. The fission-track age of a sample is computed using the classical age Equation:
(1)
where rS and rI are the spontaneous track density (determined on a non-irradiated aliquot of a sample) and the induced track density (measured on an aliquot irradiated with neutrons), respectively. l = la+lf and lf are the total decay and spontaneous fission decay constants. As la>>lf, l is assumed = la, the a-radioactivity decay constant. F is the neutron fluence, s is the cross section for the 235U induced fission by thermal neutron capture, and h is the 238U/235U isotopic abundance ratio. Equation (1) is sometimes written in different ways, according to peculiar experimental procedures or calibration systems adopted.
Since the fission-track dating early times, it was explored the potential of this new geochronological method in archaeology (Fleischer et al ., 1965a, 1965b). In principle, fission-track dating did not appear very promising for very young materials. The 238U fission half-life (~ 1016 a) is too long comparatively with mans short presence on earth: under favourable conditions only it is possible to count an adequate number of spontaneous tracks in archaeological hand-made objects. On the contrary, several authors (Suzuki, 1969; Durrani et al ., 1971; Arias Radi et al ., 1972; Bigazzi and Bonadonna, 1973; Wagner et al ., 1976) have shown that this method is an efficient alternative approach for obsidian provenance studies that can be routinely used. Application in different geographic areas such as Japan (Suzuki, 1969), Europe (Arias Radi et al ., 1972; Arias et al ., 1986a, 1986b; Bigazzi et al ., 1990), the Near East (Bigazzi et al ., 1993, 1994) proved the potentiality of this method, based on comparison of fission-track parameters (age and track densities) on artefacts and potential sources.
Although the most established techniques are based on chemical composition analyses, in some cases they do not yield an univocal answer. The fission-track dating technique is based on comparison of different parameters, so its use can potentially solve controversial results. For example, some obsidians from two important sources located in eastern Anatolia, the Bingöl volcanic field and the Nemrut Dagi volcano, are characterised by a indistinguishable chemical composition. The fission-track analysis easily discriminates these obsidians, as those from Bingöl have Pliocene ages, whereas the Nemrut Dagi obsidian flows are very recent (< 0.1 Ma). Whereas an unknown chemical composition determined on an artefact in principle does not allow any correlation, an age different from those of potential natural sources measured on an artefact may be suitable for identification of the provenance volcanic district based on geological considerations, also in areas where knowledge of characteristics of obsidian occurrences is poor.
Knowledge of South American potential sources of raw material and of circulation of obsidian in past times is rather inadequate. The subject of this work is an overview of the contribution of fission-track dating to provenance studies of obsidian in this region.
2. Short notes on fission-track dating of glass
Thermal stability of fission tracks is rather poor: a partial annealing (fading) of tracks occurs at relatively low temperatures. Equation (1) is based on the assumption that spontaneous and induced tracks are revealed with an identical efficiency, i.e . the ratio etched track present in a unit area/fissions occurred in a unit volume is assumed to be the same. Actually, partially annealed tracks are revealed with reduced efficiency. Therefore, a given fading amount produces a lowered areal track density and a proportionally lowered fission-track age. So, only in case of very fast cooling (example, volcanic rocks) a fission-track age represents a reliable formation age in minerals. Otherwise, it depends on the peculiar thermal history experienced by the rock and needs geological interpretation. For this reason, the main application field of fission-track dating is nowadays thermochronology, specially using apatite. This mineral has a closure temperature for track retention (~ 100°C) very suitable for studying low-temperature cooling histories.
Glass is a special case, as track annealing commonly occurs at room temperature also. Therefore, a fission-track age on glass is commonly a minimum age, unless a technique for correcting thermally lowered ages is applied.
Storzer and Wagner (1969) showed that track fading in glass can be detected by measuring the mean major axis of track etch pits (track size). These authors established a relationship between track size and track density reduction: smaller sizes of spontaneous versus induced track (assumed as undisturbed reference tracks) show some annealing process to have altered the tracks, producing lowered fission-track ages, commonly referred to as the apparent ages. The size-correction method is based on estimate of track density loss by track size measurements. An age correction factor is deduced using an experimental correction curve (Fig. 1).
Storzer and Poupeau (1973) proposed the plateau method, which consists in re-establishing an identical etching efficiency of spontaneous and induced tracks. Laboratory thermal treatments are imposed to a non-irradiated and to an irradiated aliquot of a sample. As partially annealed tracks are more resistant to further annealing, rS decreases less than rI. The age increases up to a plateau in which the age remains unchanged. A spontaneous to induced track size ratio, DS/DI, around 1 indicates that the plateau condition - an identical etching efficiency of spontaneous and induced tracks - is attained (Fig. 2).
Experimental evidence indicates that these techniques produce equivalent results and that size-corrected or plateau fission-track ages of glasses are commonly reliable formation ages (Arias et al ., 1981; Naeser et al ., 1980; Storzer and Wagner, 1982; Westgate, 1989), at least in case of moderate annealing and simple thermal histories. Nevertheless, the plateau method is commonly preferred, specially for its higher precision. However, in case of obsidian artefacts studies the size-correction method is a suitable alternative, as commonly identification of provenance does not require high precision ages. In addition, size measurements are very important, as the track size distribution may reveal peculiar thermal histories (examples are given below).
3. Provenance studies of South American obsidians using the fission-track method
A first significant fission-track study of South American obsidians was carried out by Miller and Wagner (1981). These authors analysed two samples from the Mullumica obsidians (Cordillera Real, east of Quito, Ecuador) and two further samples from the same region (localities of these samples is unknown to us). They dated also a Macusanite, the typical glass from the Macusani ignimbritic field (north-east Peru) as well as an obsidian from S. Lipez (Bolivia).
Miller and Wagner (1981) analysed also several artefacts from various pre-Columbian sites (Fig. 3). These are El Inga and La Esperanza, in the Quito basin, Palmar, an Ecuadorian coastal site, Alofa (in south-west Bolivia) and Cerro la Tefa/Cali (South Colombia). The results of the fission-track analysis are shown in Table 1 (for technical details see Miller and Wagner, 1981).
The very poor data-set on geological samples prevented correlation of these artefacts with specific natural sources. The artefacts from Ecuadorian sites cluster into two groups: one group with a spontaneous track density of around 500 cm-2, and the other group with a track density about ten times higher (around 5,000 cm-2). The plateau ages are slightly lower than 0.3 Ma for the first group, between 1.7 and 2 Ma for the second one. Miller and Wagner (1981) concluded that the older group did not originate from the obsidians represented by samples 041 and 042 from east of Quito, because the uranium content is different. Anyway, similarity of age indicates that the unknown source very probably is (or was) in that region. The younger source remained unidentified.
Miller and Wagner's data contain remarkable examples of the potentiality of fission-track dating. As expected, most of the obsidians showed a reduction of the DS/DI ratio. Two artefacts (075E and 080C) have a DS/DI ratio ~ 1 and an unexpected young ages (see also Fig. 4). These authors concluded that the ages of 2,060 a and 3,700 a of samples 075E and 080C dated some events of complete track annealing. By experience, a percentage of artefacts (~ 5-10 %) have the possibility of being able to reveal an archaeological age. This occurs when a thermal event produces total annealing of pre-existing tracks. Those tracks that can be etched and counted formed after the re-setting of the fission-track clock. In case of surface findings, it may be misleading to conclude that the measured age has an archaeological significance. On the contrary, when the artefact was excavated from a certain stratigraphic context, its age can be considered an archaeological age, as the annealing event is very probably correlated with its use.
A further example is artefact 080A from Cerro la Tefa, Colombia (Fig. 4c), which revealed a more complicated history. Careful analysis of the track population of this artefact revealed two populations of spontaneous tracks, one with a high track density but small size, and one with a low track density but with tracks of large size. An age correction based on the reduction of size of this second population (7.6 mm against 8.5 mm for the induced tracks) changed the measured age from 2,600 a to 3,600 a, while a plateau age determination yielded 3,400 a. This young age was interpreted to date the working of the artefact. The small tracks (average size 2.5 mm) were interpreted as those that predated a thermal event which produced a significant partial annealing of them. This population yielded a size-corrected age of 2.0 Ma, considered the geologic age of the natural source.
Table 1. Fission-track analysis of obsidians from South America
Sample | (x1015)
(cm-2) |
S
(cm-2) |
I
(cm-2) |
DS/DI | App.
Age
(Ma) |
Corr.
Age
(Ma) |
|
Geological Samples | |||||||
Peru | |||||||
Macusani 03 | 0.718 | 18,000 | 191,000 | 0.79 | 3.4 | 6.15 | |
Ecuador | |||||||
Mullumica M1 | 0.515 | 450 | 62,800 | 0.19 | |||
Mullumica M2 | 0.515 | 520 | 62,000 | 0.21 | |||
Quito 041 | 0.848 | 2,200 | 51,800 | 0.79 | 1.81 | ||
Quito 042 | 0.836 | 1,800 | 116,000 | 0.78 | 0.68 | 1.78 | |
Bolivia | |||||||
S. Lipez 046 | 1.15 | 3,800 | 199,000 | 0.76 | 2.7 | 5.09 | |
1.15 | 12,100 | 323,000 | 0.73 | 3.8 | 5.15 | ||
Artefacts | |||||||
Ecuador | |||||||
El Inga 075C | 0.836 | 4,100 | 155,000 | 1.10 | 1.72 | ||
El Inga 075D | 0.836 | 430 | 127,000 | 0.15 | |||
El Inga 075E | 0.836 | 6.3 | 126,000 | 0.98 | 0.00206 | ||
La Esperanza 078A | 0.836 | 410 | 77,400 | 0.22 | 0.21 | ||
Palmar 094A | 0.704 | 511 | 111,000 | 0.16 | |||
Palmar 094B | 0.704 | 390 | 85,000 | 0.16 | |||
Palmar 094D | 0.704 | 5,100 | 136,000 | 1.31 | 2.01 | ||
Palmar 094E | 0.704 | 410 | 101,000 | 0.14 | 0.25 | ||
Palmar 094L | 0.704 | 3,400 | 121,000 | 0.98 | |||
Palmar 094M | 0.704 | 5,000 | 117,000 | 0.15 | 0.29 | ||
Palmar 094O | 0.704 | 5,800 | 126,000 | 0.16 | |||
Palmar 094T | 0.704 | 4,100 | 115,000 | 1.25 | 1.89 | ||
Bolivia | |||||||
Alofa 079A | 0.836 | 9,900 | 119,000 | 3.5 | 6.4 | ||
Colombia | |||||||
Cerro la Tefa 080A | 0.836 | 1,000 | 160,000 | 0.36 | 0.26 | 2.0* | |
10 | 0.89 | 0.00260 | 0.0034; 0.0036* | ||||
Cerro la Tefa 080C | 1.10 | 12 | 185,000 | ~1 | 0.0037 | ||
: neutron fluence. rS: spontaneous track density. rI: induced track density. DS/DI: spontaneous to induced track size ratio. App. Age: apparent age. Corr. Age: Plateau or (*) size-corrected age. Data are presented here in a reduced form; for further details, see the original paper (after Miller and Wagner, 1981).
Arias et al . (1986a) report some age determinations for two samples found in a pyroclastic flow deposit near El Inga. At that time, they thought that those obsidians were the juvenile magma of the pyroclastic flow, however a more recent field trip suggested that they very probably are artefact splinters. Their spontaneous and induced track densities and ages, about 0.3 Ma and 2 Ma, respectively, are consistent with the two groups of artefacts identified by Miller and Wagner (1981). Correspondence and sample exchange with G.A. Wagner revealed that characteristics of glass of these two samples and of those studied by him were identical.
More recently, a detailed study of the obsidian occurrences in the Cordillera Real was published by Bigazzi et al . (1992). Three groups of obsidian occurrences have been mapped, recognised mainly by differences in their stage of preservation.
The first group includes volcanic centers that are well preserved morphologically. These volcanic centers, located in the northern part of study area (Fig. 5), show different eruptive structures.
The Mullumica and Callejones flows are monogenetic centers, both totally formed of obsidian. The Mullumica flow is a large silicic lava flow, with an estimated volume of ~ 2 Km3. The flow consists of two overlapping lobes. The basal lobe is the largest, averages 150 m thick and nearly 6 km long. The flow-lobe surface is deeply eroded, but some lateral levees and transverse ridge can still be discerned. The upper flow unit, named Corrales, forms a small squared lobe 1.5 km long, probably erupted 1 km south of the first vent. The border zone of the flows shows vertically jointed obsidian with internal textural zonation. The outer part consists of banded vesicular pumices and glassy obsidian, brecciated near the surface. The central part of the flow consists mainly of black obsidian with spherulites and flow-banded perlitic obsidian.
The Callejones flow is smaller but otherwise similar to the Mullumica, consisting of a flow 3 km long with an estimated volume of ~ 0.5 Km3. Its state of preservation and lithology suggest that that erupted close to the time of Mullumica eruption. North of the Callejones flow is a small outcrop of obsidian blocks called Yurac Paccha.
The Potrerillos volcano is a small polygenetic center with obsidian occurring only in late breccia deposits close to the vent.
The second group is composed of obsidian outcrops that probably belong to different lava flow-units which were closely associated in space and time, and were probably erupted from a multi-vent center. Although these obsidian flows are deeply eroded by glaciers and cut by Inter-Andean Depression border faults, the original lava field can still be reconstructed. In addition, the grade of devitrification of the obsidian rocks is similar in all outcrops.
El Tablon is an ancient obsidian lava flow cropping out on the west flank of the Cordillera Real. It is deeply cut by the Pifo glacial valley and faulted by border faults of the Inter-Andean Depression (Fig. 5). A very similar obsidian is exposed on the northern side of the Pifo Valley and on Loma Pishanga. These obsidian flows have a volume of ~ 4 km3. The obsidian body consists of perlitised obsidian bedded with completely hydrated material, forming platy and vertically jointed walls.
The third group consists of erosional remnants of obsidian flows in the upper part of the oldest volcanic succession in the region, the Basal Volcanic Complex (BVC) of Barberi et al . (1988). These obsidians cropping out on Loma Quiscatola and Cerro Yanaurcu outcrops, occur as breccia deposits with crystallised matrix; neither the original surface morphology nor shape of the flows is preserved. The Rodeo Corrales obsidian blocks belong to a secondary deposit, near Cerro Yanaurcu, that contains a high percentage of fresh glassy obsidian; it represents an ancient debris flow deposit, subsequently cut by a glacial valley.
In the Ecuadorian highlands, obsidian has been reported from paleo-Indian sites dating back to 9,000 - 10,000 years ago. Most sites are located in the Ilaló region, a short distance east of Quito, close to the potential natural sources of these raw materials studied by Bigazzi et al . (1992).
Archaeological research carried out at the Mullumica and Quiscatola outcrops has found evidence of quarrying in pre-Colombian times, from 3477 BC to the time of the Inca invasion ( ca . 1460 AD).
For further details on the geological setting of the obsidian occurrences described here and on their geo-archaeological significance, see Bigazzi et al ., 1992.
Table 2. Fission-track analysis of obsidians from the Cordillera Real, east of Quito (Ecuador)
Sample | (x1015)(cm-2) | S (cm-2) | nS | I (cm-2) | nI | DS/DI | Age
(± 1s)
(Ma) |
heating | |
1. Mullumica flow, | 1.61 C | 440 | 111 | 244,000 | 1,054 | 0.96 | 0.17 ± 0.02 | ||
Laguna Yanacoche | 220 | 82 | 104,000 | 1,374 | 1.00 | 0.20 ± 0.03 | 3h 300°C | ||
2. Mullumica upper | 1.61 C | 440 | 139 | 243,000 | 1,433 | 0.95 | 0.18 ± 0.02 | ||
flow, CH 2010 | 215 | 71 | 116,000 | 1,204 | 1.01 | 0.18 ± 0.03 | 2h 250°C | ||
3. Mullumica flow, | 1.61 C | 420 | 163 | 26,000 | 2,180 | 0.91 | 0.15 ± 0.02 | ||
Abrigo 2 | 395 | 154 | 201,000 | 2,416 | 1.03 | 0.19 ± 0.02 | 2h 200°C | ||
4. Mullumica flow, | 1.63 P | 430 | 150 | 261,000 | 1,024 | 0.96 | 0.16 ± 0.01 | ||
Abrigo 26 de Mayo | 295 | 158 | 161,000 | 1,259 | 1.03 | 0.18 ± 0.02 | 2h 250°C | ||
5 . Callejones flow, | 1.63 P | 400 | 174 | 239,000 | 1,495 | 0.97 | 0.16 ± 0.01 | ||
sample 2 | 283 | 198 | 160,000 | 1,253 | 0.97 | 0.17 ± 0.01 | 2h 250°C | ||
6. Callejones flow, | 1.61 C | 355 | 91 | 204,000 | 1,935 | 0.95 | 0.17 ± 0.02 | ||
sample 3 | 205 | 104 | 87,000 | 1,399 | 1.00 | 0.22 ± 0.02 | 3h 300°C | ||
7. Potrerillos volcano | 1.40 C | 255 | 23 | 113,000 | 597 | 1.05 | 0.19 ± 0.05 | ||
8. Yurac Paccha | 1.63 P | 690 | 66 | 199,000 | 1,298 | - | 0.34 ± 0.04 | ||
395 | 45 | 101,000 | 365 | - | 0.39 ± 0.06 | 2h 250°C | |||
9. Rodeo Corrales | 1.63 P | 3,680 | 416 | 355,000 | 1,122 | 0.74 | 1.01 ± 0.06 | ||
3,080 | 321 | 215,000 | 1,367 | 0.98 | 1.38 ± 0.09 | 2h 250°C | |||
10. Cerro Yanaurcu | 1.63 P | 4,600 | 560 | 369,000 | 1,460 | 0.87 | 1.22 ± 0.06 | ||
3,440 | 628 | 221,000 | 1,052 | 1.02 | 1.52 ± 0.08 | 2h 250°C | |||
11. El Tablon flow, | 1.40 C | 1,400 | 421 | 180,000 | 2,725 | 0.89 | 0.66 ± 0.06 | ||
Loma Pishanga | 1,110 | 333 | 110,000 | 2,003 | 1.00 | 0.84 ± 0.08 | 2h 200°C | ||
CH 577 (*) | 0.157 P | 732 | 150 | 11,800 | 611 | 0.84 | 0.60 ± 0.05 | ||
Size Corrected age | 0.81 ± 0.08 | ||||||||
12. Loma Quiscatola, | 1.63 P | 4,870 | 846 | 420,000 | 1,328 | 0.76 | 1.13 ± 0.05 | ||
CH 2027 | 4,070 | 601 | 237,000 | 1,131 | 0.97 | 1.68 ± 0.09 | 2h 250°C | ||
4,010 | 1,045 | 238,000 | 1,327 | 1.02 | 1.64 ± 0.07 | 4h 250°C | |||
nS (nS): spontaneous (induced) track counted. Heating: thermal treatment imposed for plateau age determination. Both the Campinas (C) and Pisa (P) fission-track groups analysed all samples. A selection of data is shown here. For further details, see the original paper (after Arias et al ., 1986a (*), and Bigazzi et al ., 1992).
Analytical results are shown in Table 2 (for technical details, see Bigazzi et al ., 1992). Three groups of ages can be recognised for the obsidian rocks studied:
(1) Very young obsidians, slightly less than 0.2 Ma old, were sampled from the Mullumica and Callejones flows and the Potrerillos volcano. A K-Ar age of 0.09±0.03 Ma is also available from a dacite sample from the Potrerillos volcano (Barberi et al ., 1988).
(2) Intermediate-age obsidians, with ages around 0.4 Ma for the Yurac Paccha sample and about 0.85 Ma for the Loma Pishanga sample. The latter age confirms that the sample is from the rocks of the El Tablon lava field, for which a fission-track age of 0.81 Ma (sample CH 577) had been determined. A K-Ar of 0.81 Ma is also available (Barberi et al ., 1988).
(3) Older obsidians, with ages between 1.4 and 1.7 Ma; they come from outcrops located in the upper part of BVC: Cerro Yanaurcu, Rodeo Corrales and Loma Quiscatola.
Results regarding Ecuadorian obsidians in Tables 1 and 2 show excellent agreement.
Table 3. Fission-track parameters of obsidian artefacts from two Pacific coastal sites
Sample | S
(cm-2) |
I
(x 103)
(cm-2) |
DS/DI | App.
Age
(Ma) |
Plateau Age
(Ma) |
|
La Tolita (Ecuador) | ||||||
LT1, 2, 3, 4, 7 and 8 | 270-860 | 135-244 | 0.66-0.94 | 0.10-0.21 | 0.24-0.31 | |
LT5 | 3,240 | 182 | 0.85 | 1.07 | 1.65 | |
LT 6 (*) | 720 | 103 | 1.01 | 0.42 | ||
Inguapi (Colombia) | ||||||
I1 | 580 | 192 | 0.81 | 0.18 | 0.25 | |
I2, 3 | 5,100-6,580 | 160-221 | 0.81-0.88 | 1.78-1.91 | 1.58-2.60 | |
The induced track density, rI, is normalised to a neutron fluence of 1015 cm-2, as artefacts were irradiated with different fluences. (*): track densities and track-size ratio refer to the plateau age determination, as only these data are available for this artefact. Several determinations by two observers were made by the Grenoble fission-track group. Data are presented here in a synthetic form; for further details, see the original paper (after Dorighel et al ., 1994).
Comparison of archaeological artefacts with natural sources can be a puzzling task. Spontaneous and induced track densities and ages of those studied by Arias et al . (1986a) suggest that none of the outcrops represented by samples 1 through 12 of Table 2 can be the source of them.
Analytical data for some of the artefacts reported in Miller and Wagner (1981) are similar to data for the young obsidians as well as the older obsidians of Table 2. However, correlation of artefacts with natural sources can be safely obtained by comparison of the fission-track parameters combination. Some of them (for example, the track densities) depend, at least partially, on the technique adopted (etching conditions, counting criteria). Therefore, it could be misleading to base provenance identifications on fission-track data obtained by different laboratories. For this reason, one should not attempt to assign these artefacts reported in Miller and Wagner (1981) to specific sources represented by samples shown in Table 2.
Another South American volcanic area on which detailed geochronological investigation was carried out is the ignimbritic field of Macusani. Macusanite is a peculiar glass which, before Miller and Wagner (1981), had been dated for the first time as soon as the fission-track dating method was invented (Fleischer and Price, 1964). Macusanite rounded pebbles are found in river banks and fluvio-glacial deposits inside and around the ignimbritic field. As this glass was proposed as a potential age standard (McCorkell and Naeser, 1988), several samples from different valleys were recently analysed (Poupeau et al ., 1992, 1993). Formation ages of Macusanites cluster into two groups, around 5.6 Ma and around 7 Ma. These glasses are very transparent, sometimes pale green or pale yellow coloured. The peculiar characteristics of Macusanite, including a high spontaneous fission-track density (16,000-30,000 cm-2) in comparison with other obsidians, make it a unique glass that can be very easily discriminated from other glasses.
A provenance study of obsidian artefacts from two sites, La Tolita (North Ecuador) and Inguapi (South Colombia), was published by the fission-track group of Grenoble, France (Dorighel et al . (1994). These sites are located in the Pacific coastal area which had a very important cultural development during pre-Columbian times, between 700-600 BC and around 300 AD.
The artefacts from La Tolita and Inguapi belong to four groups of age (Table 3; for technical details see Dorighel et al ., 1994). The main group (plateau-age between 0.25 Ma and 0.30 Ma) closely recalls the group characterised by an age of around 0.30 Ma identified at Palmar by Miller and Wagner (1981) and recognised also by Bigazzi et al . (1986a, 1992). The fission-track parameters of artefact LT5 point to the older occurrences studied by the latter authors. Artefact LT6 also has an age consistent with obsidians from the same area, while artefacts I2 and I3 do not recall any previously analysed source.
The results obtained by Dorighel et al . (1994) confirm that most of the artefacts from the coastal sites of the Formative Period (3500-300 BC) as well as of the Regional Development Period (300 BC - 500 AD) originated from the volcanic field described above.
4. New fission-track analyses
The Italian and Brazilian fission-track groups involved in this research on South American glasses collaborate with the Grenoble group with exchange of data and information as well as samples. Several new analyses are already available or in progress.
An overview of the recent research carried out at Grenoble will be presented at the 49 ICA (Dorighel et al ., 1997). It will include age determinations of artefacts from several coastal sites and sites from the highlands of Ecuador and Colombia which confirm the importance of the unknown source dated 0.25-0.30 Ma. Most artefacts originated from it. Two artefacts are correlated with the Yanaurcu-Quiscatola obsidians. These authors dated the Rio Hondo (Colombia) obsidian, and identified this in the nearby archaeological site La Elvira.
Age determinations on obsidians from the Cotopaxi volcano (Fig. 3) are now available. the Cotopaxi volcano, located in the middle portion of the Inter-Andean Depression, is the highest active volcano on the earth. It is well known for the magnificence of its cone topped by ice and for its very dangerous historical eruptions. Barberi et al . (in preparation) have outlined the entire eruptive history of the Cotopaxi volcanic complex. A more ancient volcano, named Palaeo-Cotopaxi, was initially characterised by large explosive eruptions during which rhyolitic Plinian falls and ash-flows were deposited. Following the early strong explosive phase, an andesitic mild explosive and effusive eruption activity took place to build up a small stratovolcano slightly to the south of the present cone.
After a long-lasting erosive period, volcanic activity resumed in the Cotopaxi area producing three large rhyolitic Plinian eruptions. A cone-building phase characterised by emission of andesitic lava and pyroclastic material followed. The growth of the cone was interrupted by a slope failure producing a giant debris avalanche that flowed in the Rio Pita Valley.
Obsidian blocks were sampled in the pyroclastic ash flow deposits of the Plinian eruptions of the early strong explosive phase of both Palaeo-Cotopaxi and Cotopaxi. Obsidian glass is rhyolitic in composition and include rare biotite and quartz crystals. Sample CTX 46 (Table 4) belongs to the first Plinian eruption of the Palaeo-Cotopaxi volcano and CTX 45 to the following one. Samples CTX 67 and CTX 92 were collected from the ash flow deposits of the second Plinian eruption of Cotopaxi and sample CTX 73 from the following one.
Table 4. Fission-track analysis of obsidians from the Cotopaxi Volcano (Ecuador)
Sample
heating |
(x1015)
(cm-2) |
S (cm-2) | nS | I (cm-2) | nI | DS/DI | Age
(± 1s)
(Ma) |
|
CTX 45 | 3.19 | 1,020 | 492 | 438,000 | 1,271 | 0.83 | 0.45 ± 0.03 | |
3h 250°C | 860 | 276 | 293,000 | 1,703 | 1.00 | 0.56 ± 0.04 | ||
CTX 46 | 3.19 | 770 | 212 | 253,000 | 1,365 | 0.85 | 0.42 ± 0.03 | |
3h 250°C | 750 | 126 | 264,000 | 1,279 | 1.00 | 0.54 ± 0.05 | ||
CTX 67 | 3.19 | 620 | 82 | 383,000 | 740 | 0.88 | 0.31 ± 0.04 | |
CTX 73 | 3.19 | 25 | 11 | 261,000 | 1,134 | 0.94 | 0.018 ± 0.006 | |
CTX 92 | 3.19 | 9 | 2 | 202,000 | 1,169 | 0.008 ± 0.006 | ||
The neutron fluence, , is referred to the SRM 962a NIST glass standard, using as reference the mean of Cu and Au NIST calibrations; the irradiation with thermal neutrons was performed in the Lazy Susan facility, Cd ratio 6.4 for Au and 48 for Co, of the LENA Triga Mark II reactor of the University of Pavia, Italy. Parameters used for age calculation: l = 1.55125 x 10-10 a-1; lF = 7.03 x 10-17 a-1; s = 5.802 x 10-22 cm2; 238U/235U = 137.88. Error of age includes propagation of Poisson counting errors.
These new ages, which confirm the potential of fission-track dating also in case of very young glasses, contribute to a better knowledge of the chronological evolution of the volcanism of the Andean region. By the archaeometric point of view, the fission-track parameters fully discriminate the Cotopaxi obsidians from those shown in Table 2. None of the artefacts previously analysed appear to have been originated from the Cotopaxi volcano.
Finally, six artefacts from three sites - Real Alto (Guayas), Pechiche (Guayas) and San Isidro (Manabí) - were very recently studied. The six artefacts belong to two distinct groups (formation ages 1.5-1.7 Ma, and ~ 0.2 Ma, respectively). Comparison of fission-track parameters allow to correlate them with the Loma Quiscatola and Mullumica obsidians, These analyses will be presented in detail at the 49 ICA (Marcos et al ., 1997).
5. Conclusions
Research performed in co-operation with archaeologists and Geologists by different fission-track groups on South American obsidians contributed to fill up blanks concerning characterisation of potential sources of raw material and provenance identification of obsidian artefacts. However, knowledge of obsidian occurrences in South America is still inadequate. Besides those quoted here, very few further data are available, sometimes for samples of uncertain provenance.
The best known region is the central part of the Cordillera Real. The obsidian rhyolitic volcanism has been active during the entire Quaternary in this area, but was concentrated in three fairly short episodes. The oldest, belonging to the end of BVC, forms the basement for the Quaternary stratovolcanoes and fixes the upper age limit around 1.5 Ma. This limit is in close agreement with the period of increasing volcanic activity, about 1.5-1.0 Ma, that marks the birth of the actual volcanic arc, according to Barberi et al . (1988). More recent episodes of obsidian rhyolitic volcanism correspond to the activity of the present volcanic arc.
Most artefacts found in pre-Columbian sites located along the Pacific coast or in the highlands in Ecuador and South Colombia originated from these obsidian occurrences. Numerous of them form a group with an age of 0.25-0.30 Ma. Characteristics of these samples do not fit with any specific know source. However, these artefacts (1) were found in association with other artefacts more referable to the Cordillera Real, (2) also in sites located in that area, and (3) their age is well consistent with this volcanism. These factors allow to conclude that the unknown source belongs to these obsidian occurrences. A second group is formed by older artefacts (1.7-2.0 Ma). Whereas several of them show fission-track parameters which only generically point to the oldest obsidians of the Cordillera Real, some artefacts are well correlated with specific occurrences (Quiscatola, Yanaurcu). A small group of artefacts originated from the Mullumica obsidians.
Finally, the identification made of glass from the Cordillera Real in the Real Alto coastal site (south-west Ecuador), where obsidian blades were found in association with Valdivia 7 pottery (1850-1750 BC), gives a new evidence of an early long-distance trade between the Quito basin and the Santa Elena Peninsula.
Although characteristics of potential natural sources of raw material were reasonably studied in some areas of South America only, it could be misleading to conclude that an exhaustive knowledge of the existing obsidian occurrences will solve all provenance identification problems. Significant morphological changes were produced by natural events and human activities in the last millennia which may have made inaccessible ancient sources. Some of them could be covered by recent alluvium, other could have been exhausted by prehistoric man, as documented for other regions of the earth, for example eastern Slovakia (Bigazzi et al ., 1990).
Acknowledgements . We wish to thank L. Bellot-Gurlet, O. Dorighel, E. Labrin and G. Poupeau (Grenoble) and Á. Alvarez and J.G. Marcos (Barcelona) who contributed to this work with helpful suggestions and made available also unpublished data.
References
Arias C., Bernardes C., Bigazzi G., Bonadonna F.P., Cesar M.F., Hadler Neto J.C., Lattes C.M.G., Oliveira J.X., Osorio Araya A.M., Radi G. (1986a) Identificação de provenência de manufaturados de obsidiana através da datação com o método do traço de fissão. Ciência e Cultura , 38 , 285-308.
Arias C., Bigazzi G. and Bonadonna F.P. (1981) Size correction and plateau age in glass shards. Nucl. Tracks Radiat. Meas ., 5 , 129-136.
Arias C., Bigazzi G., Bonadonna FP., Cipolloni M., Hadler J.C., Lattes C.M.G. and Radi G. (1986b) Fission track dating in archaeology. A useful application. In : Scientific Methodologies Applied to Works of Art . Edited by P.L. Parrini. Montedison, Progetto Cultura Eds., Milan, Italy, 151-159.
Arias-Radi G., Bigazzi G. and Bonadonna FP. (1972) Le tracce di fissione. Un metodo per lo studio delle vie di commercio dell'ossidiana. Origini , 6 , 155-170.
Barberi F., Coltelli M., Ferrara G., Innocenti F., Navarro J.M. and Santacroce R. (1988) Plio-Quaternary volcanism in Ecuador. Geological Magazine , 125 , 1-14.
Bigazzi G. and Bonadonna FP. (1973) Fission track dating of the obsidian of Lipari Island (Italy). Nature , 242 , 322-323.
Bigazzi G., Coltelli M., Hadler N. J.C., Osorio Araya A.M., Oddone M. and Salazar E. (1992) Obsidian bearing lava flows and pre-Columbian artefacts from Ecuadorian Andes: first new multidisciplinary data. J. South Am. Earth Sci ., 6 , 21-32.
Bigazzi G., Ercan T., Oddone M., Özdogan M. and Yegingil Z. (1993) Application of fission track dating to archaeometry: provenance studies of prehistoric obsidian artefacts. Nucl. Tracks Radiat. Meas. , 22 , 757-762.
Bigazzi G., Marton P., Norelli P. and Rozloznik L. (1990) Fission track dating of Carpathian obsidians and provenance identification. Nucl. Tracks Radiat. Meas., 17 , 391-398.
Bigazzi G., Yegingil Z., Ercan T., Oddone M. and Özdogan M., (1994) Provenance studies of prehistoric artefacts in Eastern Anatolia: interdisciplinary research results. Miner. Petrogr. Acta , XXXVII , 17-36.
Dorighel O., Bellot-Gurlet L. and Poupeau G.(1997) Caracterizacion de artefactos en obsidiana mediante PIXE y Trazas de Fision : un enfoque sobre las fuentes de materia prima utilizadas en Ecuador y Colombia entre 3500 AC y 1500AD. 49 ICA, International Congress of Americanists, 7-11 July 1997, Quito, Ecuador. Proceedings.
Dorighel O., Poupeau G., Bouchard J.-F. and Labrin E. (1994) Datation par traces de fission et étude de provenance dartefacts en obsidienne des sites archéologiques de La Tolita (Equateur) and Inguapi (Colombie). Bull. Soc. Préhist. Fr ., 91 , 133-144.
Durrani S.A., Khan H.A., Taj M. and Renfrew C. (1971) Obsidian source identification by fission track analysis. Nature , 233 , 242-245.
Fleischer R.L. and Price P.B. (1964) Fission track evidence for the simultaneous origin of tektites and other natural glasses. Geochim. Cosmichim. Acta , 28 , 755-760.
Fleischer R.L., Price P.B., Walker R.M. and Leakey L.S.B. (1965a) Fission track dating of bed I, Olduvai Gorge. Science , 148 , 72-74.
Fleischer R.L., Price P.B., Walker R.M. and Leakey L.S.B. (1965b) Fission track dating of a mesolithic knife. Nature , 205 , 1138.
Marcos J. G., Álvarez A. and Bigazzi G. (1997) El Tráfico a Distancia Temprano entre La Hoya de Quito y la Península de Santa Elena: Las evidencias de Real Alto. 49 ICA, International Congress of Americanists, 7-11 July 1997, Quito, Ecuador. Proceedings .
Miller D.S. and Wagner G.A. (1981) Fission-track ages applied to obsidian artefacts from South America using the plateau-annealing and track-size age-correction techniques. Nucl. Tracks Radiat. Meas ., 5 , 147-155.
McCorkell R. and Naeser C.W. (1988) Natural glass inter-laboratory reference materials for fission-track dating. 6th Int. Workshop on Fission-Track Dating, 5-9 Sept. 1988, Besançon, France. Abstract, 1p. Reproduced in Nucl. Tracks Radiat. Meas ., 17 , 422.
Naeser C.W., Obradovich J.D. and Izett G.A. (1980) Fission-track and K-Ar ages of natural glasses. Geological Survey Bulletin , 1489, 32p.
Poupeau G., Labrin E., Sabil N., Bigazzi G., Arroyo P.G. and Vatin-Perignon N. (1993) Fission track dating of 15 Macusanite glass pebbles from the Macusani volcanic field (SE Peru). Nucl. Tracks Radiat. Meas ., 21 , 499-506.
Poupeau G., Sabil N., Villa I.M., Bigazzi G., Vatin-Perignon N., Flores P., Pereyra P., Salas P. and Arroyo G. (1992) Fission-track and K-Ar ages of "Macusanite" obsidian glasses, (SE Peru): geodynamic implications. Tectonophysics , 205 , 295-305.
Price P.B. and Walker R.M. (1962) Chemical etching of charged-particle tracks in solids. J. Appl. Phys ., 33 , 732-734.
Price P.B. and Walker R.M. (1963) Fossil tracks of charged particles in mica and the age of minerals. J. Geophys. Res ., 68 , 4847-4862.
Storzer D. and Poupeau G. (1973) Ages-plateau de minéraux et verres par la méthode des traces de fission. C. R. Acad. Sci. Paris , 276 serie D , 317-319.
Storzer D. and Wagner G.A. (1969) Correction of thermally lowered fission track ages of tektites. Earth Planet. Sci. Lett ., 5 , 463-468.
Storzer D. and Wagner G.A. (1982) The application of fission track dating in stratigraphy: a critical review. In: Numerical dating in stratigraphy . Edited by G.B. Odin, John Wiley & sons Ltd. Eds., 199-222.
Suzuki M. (1969) Fission track dating and uranium contents of obsidians. Daiyonki Kenkyu , 8 , 123-130.
Wagner GA, Storzer D. and Keller J. (1976) Spaltspurendatierung quartärer Gesteinsgläser aus dem Mittelmeerraum. N. Jb. Miner. Mh ., 2 , 84-94.
Wagner G.A. and Van den haute P. (1992) Fission-track dating . Kluver Academic Publishers Eds., Dordrecht, The Netherlands, 285p.
Westgate J.A. (1989) Isothermal plateau fission track ages of hydrated glass shards from silicic tephra beds. Earth Planet. Sci. Lett., 95 , 226-234.
CAPTIONS
Fig. 1. Size-correction curve for the Mt. Arci obsidian, Sardinia (Italy). On an irradiated aliquot of glass a variable amount of artificial annealing of the induced tracks was produced by various thermal treatments at temperatures between 200°C and 350°C. Each experimental point represents the size reduction (D/Do) and the decrease of track areal density (r/ro) measured after a given thermal treatment, assuming as reference size (Do) and density (ro) determined on an unheated fraction of the glass. The resulting curve was used for correcting the apparent age of this obsidian. By the spontaneous to induced track size ratio, DS/DI, it was deduced a correction factor (C.F.): the 'true' age of the sample was obtained by changing (rS/rI) with (rS/rI)/ C.F. in Equation (1).
Fig. 2. Plot of data to illustrate the plateau technique. (a): Plateau fission-track age determination on an obsidian from Sarikamis, eastern Anatolia. Each experimental point represents a determination made after cumulative thermal treatments of two hours at the temperatures indicated in abscissa. For example, the first points on the left refer to the natural sample, whereas the last on the right were determined on the sample after a thermal treatment of 2 h at 100°C + 2 h at 150°C + 2 h at 200°C + 2 h at 250°C. The induced track sizes (as well as areal density) reduce more quickly than the spontaneous track sizes (and density). In the plateau region the spontaneous to induced track size ratio is ~ 1. (b) illustrates the plateau age determined by Miller and Wagner (1981) on artefact 094M from Palmar, an Ecuadorian pre-Columbian coastal site. In this case these author plot age and track density reduction: rS (rI) refers to spontaneous (induced) tracks, ro is the original track density. The plateau condition is attained when spontaneous and induced tracks suffered an identical annealing amount, detected by an identical track areal density reduction (Fig. 2b has been redrawn after Miller and Wagner, 1981).
Fig. 3. Schematic map showing location of pre-Columbian sites of Ecuador and South Colombia considered for provenance studies of obsidian artefacts using the fission-track analysis. The obsidian occurrences potential sources of raw material for tool-making of the central sector of the Cordillera Real was studied in detail by Bigazzi et al . (1992). The shaded area represents their study area, reported in detail in Fig. 5.
Fig. 4. Track-size distributions of obsidian from South America. The analysis of the track-size distribution is an efficient tool for deciphering thermal histories of natural glasses. Commonly obsidians show a reduction of the sizes of the spontaneous tracks in comparison with those of the induced tracks, assumed as reference of undisturbed tracks. This is due to the low stability of fission-tracks during geological times: a certain amount of annealing occurs at room temperatures also. This is the case of the obsidian from Cerro Yanaurcu (a), Ecuador (DS/DI = 0.87, Table 2). Two artefacts from Ecuadorian sites studied by Miller and Wagner (1981) revealed complex thermal histories. In b) a DS/DI value ~ 1 indicates that spontaneous tracks are unaffected by partial annealing. An unexpected young age was determined on this sample (2,060 a, Table 1). Miller and Wagner (1981) concluded that artefact 075E experienced a heating event occurred during its use that completely erased pre-existing tracks. The tracks observed by them were due to fissions occurred afterwards. Therefore, the determined age was an archaeological age, because it dated the use of the artefact. Artefact 080A is a rare example of bimodal distribution of sizes of spontaneous tracks. In this case the thermal event was not strong enough to completely erase already accumulated tracks. From the two track populations, characterised by smaller tracks and larger tracks, respectively, it was possible to determine the formation age of the glass (geological age) as well as the age of the use of the artefact (Fig. 3b and 3c have been redrawn after Miller and Wagner, 1981).
Fig. 5. Geological sketch map of the area in the Cordillera Real east of Quito studied by Bigazzi et al ., 1992. Numbered + signs indicate the location of samples of Table 2; a: undifferentiated basal volcanic complex; b: volcaniclastic deposits filling the Inter-Andean Depression; c: metamorphic basement of the Cordillera Real; d: volcanic products of the Antisana stratovolcano; e: andesitic lava flows (Antisanilla and Papallacta are historical lava flows); f: obsidian lava flows (the inferred boundary of the El Tablon flow is shown by dashed line); g: non-vitrophyric silicic volcanic centres; h, inferred normal faults of Inter-Andean Depression; i: Cordillera Real watershed; l: eruptive vents and craters (after Bigazzi et al ., 1992).
SIMPOSIO: ARQ 14
"ARQUEOMETRIA, LA TRANSFORMACION DEL DATO ARQUEOLOGICO EN EVIDENCIA DE FORMACIONES SOCIALES EN LA ARQUEOLOGIA"
Simposio en Memoria de José Luis Lorenzo
Organizadores :
Jorge G. Marcos
Aurelio Alvarez Pérez
PONENCIA:
Provenance Studies of Obsidian Artefacts Using the Fission-Track Analysis in South America: an Overview
(Estudios de Areas Fuente de Artefactos de Obsidiana, usando el Análisis de
Trazas de Fisión en Sur América)
By Giulio Bigazzi1, Mauro Coltelli2, Julio Cesar Hadler Neto3 and Ana Maria Osorio Araya4
1C.N.R. Istituto di Geocronologia e Geochimica Isotopica, Via Cardinale Maffi 36, 56127 Pisa, Italy.
2C.N.R. Istituto Internazionale di Vulcanologia, Piazza Roma 2, 95123 Catania, Italy.
3Dep.to Raios Cosmicos, Instituto de Fisica, Universidade Estadudal de Campinas - UNICAMP
C.P. 6165, 13081 Campinas SP, Brazil.
4Dep.to de Ciências Ambientais, Universidade Estadual Paulista - UNESP
C.P. 957, 19060 Presidente Prudente SP, Brazil
Correspondence:
Giulio Bigazzi - Istituto di Geocronologia e Geochimica Isotopica, CNR
Via Cardinale Maffi, 36, 56127 Pisa (Italy)
Ph: **39-(0)50-560110. Fax: **39-(0)50-900270. E-mail: iggi@iggi.pi.cnr.it
Abstract
Application of the fission-track dating method to glass samples and its use for provenance studies of obsidian artefacts is briefly described. The results obtained by various fission-track groups which studied South American obsidians in the last fifteen years are presented. Only few obsidian-bearing volcanic fields have been studied in detail. Among them, the volcanics located in the central sector of the Cordillera Real, east of Quito, Ecuador, appear to have been an important source area of raw material for tool-making during pre-Columbian times. Artefacts from archaeological sites located along the coast of Ecuador and southern Colombia or in the Inter-Andean Depression cluster into two main groups of age (0.25-0.30 Ma and 1.7-2 Ma). Although the fission-track parameters identify the Cordillera Real as source area, in few cases only they allow correlation with specific known obsidian occurrences (Mullumica, Loma Quiscatola). The identification made of glass from the Cordillera Real in the Real Alto coastal site (south-west Ecuador), where obsidian blades were found in association with Valdivia 7 pottery (1850-1750 BC), gives a new evidence of an early long-distance trade between the Quito basin and the Santa Elena Peninsula.
Buscar en esta seccion :